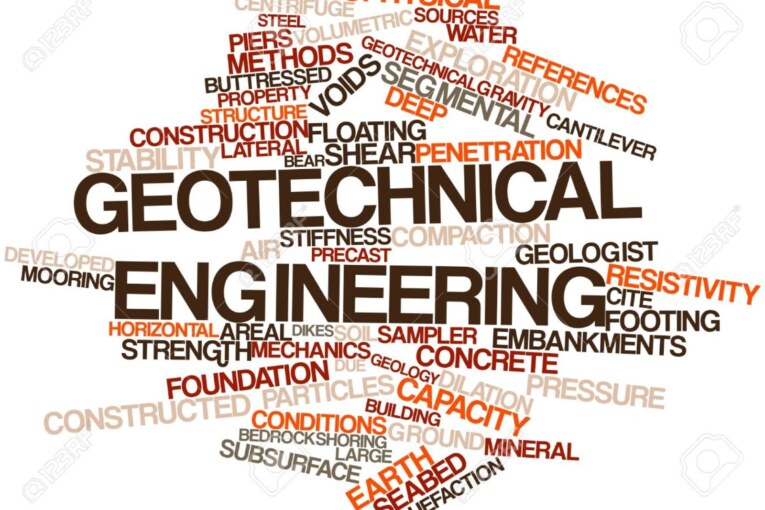
Geotechnical engineering is the branch of civil engineering concerned with the engineering behavior of earth materials. Geotechnical engineering is important in civil engineering, but also has applications in military, mining, petroleum and other engineering disciplines that are concerned with construction occurring on the surface or within the ground. Geotechnical engineering uses principles of soil mechanics and rock mechanics to investigate subsurface conditions and materials; determine the relevant physical/mechanical and chemical properties of these materials; evaluate stability of natural slopesand man-made soil deposits; assess risks posed by site conditions; design earthworks and structure foundations; and monitor site conditions, earthwork and foundation construction.
Soil mechanics
In geotechnical engineering, soils are considered a three-phase material composed of: rock or mineral particles, water and air. The voids of a soil, the spaces in between mineral particles, contain the water and air.
The engineering properties of soils are affected by four main factors: the predominant size of the mineral particles, the type of mineral particles, the grain size distribution, and the relative quantities of mineral, water and air present in the soil matrix. Fine particles (fines) are defined as particles less than 0.075 mm in diameter.
Soil Properties
Some of the important properties of soils that are used by geotechnical engineers to analyze site conditions and design earthworks, retaining structures, and foundations are:
- Specific weight or Unit Weight: Cumulative weight of the solid particles, water and air of the unit volume of soil. Note that the air phase is often assumed to be weightless.
- Porosity: Ratio of the volume of voids (containing air, water, or other fluids) in a soil to the total volume of the soil. Porosity is mathematically related to void ratio.
- Void ratio: The ratio of the volume of voids to the volume of solid particles in a soil mass.
- Permeability: A measure of the ability of water to flow through the soil. It is expressed in units of velocity.
- Compressibility: The rate of change of volume with effective stress. If the pores are filled with water, then the water must be squeezed out of the pores to allow volumetric compression of the soil; this process is called consolidation.
- Shear strength: The maximum shear stressthat can be applied in a soil mass without causing shear failure.
- Atterberg Limits: Liquid limit, Plastic limit, and Shrinkage limit. These indices are used for estimation of other engineering properties and for soil classification.
Building foundations
The primary considerations for foundation support are bearing capacity, settlement, and ground movement beneath the foundations. Bearing capacity is the ability of the site soils to support the loads imposed by buildings or structures. Settlement occurs under all foundations in all soil conditions, though lightly loaded structures or rock sites may experience negligible settlements. For heavier structures or softer sites, both overall settlement relative to unbuilt areas or neighboring buildings, and differential settlement under a single structure, can be concerns. Of particular concern is settlement which occurs over time, as immediate settlement can usually be compensated for during construction. Ground movement beneath a structure’s foundations can occur due to shrinkage or swell of expansive soils due to climatic changes, frost expansion of soil, melting of permafrost, slope instability, or other causes. All these factors must be considered during design of foundations.
Shallow Foundation
Shallow foundations are a type of foundation that transfers building load to the very near the surface, rather than to a subsurface layer. Shallow foundations typically have a depth to width ratio of less than 1.
Footings
Footings (often called “spread footings” because they spread the load) are structural elements which transfer structure loads to the ground by direct areal contact. Footings can be isolated footings for point or column loads, or strip footings for wall or other long (line) loads. Footings are normally constructed from reinforced concrete cast directly onto the soil, and are typically embedded into the ground to penetrate through the zone of frost movement and/or to obtain additional bearing capacity.
Slab Foundations
A variant on spread footings is to have the entire structure bear on a single slab of concrete underlying the entire area of the structure. Slabs must be thick enough to provide sufficient rigidity to spread the bearing loads somewhat uniformly, and to minimize differential settlement across the foundation. In some cases, flexure is allowed and the building is constructed to tolerate small movements of the foundation instead. For small structures, like single-family houses, the slab may be less than 300 mm thick; for larger structures, the foundation slab may be several meters thick.
Deep Foundations
Deep foundations are used for structures or heavy loads when shallow foundations cannot provide adequate capacity, due to size and structural limitations. They may also be used to transfer building loads past weak or compressible soil layers. While shallow foundations rely solely on the bearing capacity of the soil beneath them, deep foundations can rely on end bearing resistance, frictional resistance along their length, or both in developing the required capacity. Geotechnical engineers use specialized tools, such as the cone penetration test, to estimate the amount of skin and end bearing resistance available in the subsurface.
Offshore geotechnical engineering
Offshore (or marine) geotechnical engineering is concerned with foundation design for human-made structures in the sea, away from the coastline (in opposition to onshore or nearshore). Oil platforms, artificial islands and submarine pipelines are examples of such structures. There are number of significant differences between onshore and offshore geotechnical engineering. Notably, ground improvement (on the seabed) and site investigation are more expensive, the offshore structures are exposed to a wider range of geohazards, and the environmental and financial consequences are higher in case of failure. Offshore structures are exposed to various environmental loads, notably wind, waves and currents. These phenomena may affect the integrity or the serviceability of the structure and its foundation during its operational lifespan – they need to be taken into account in offshore design.